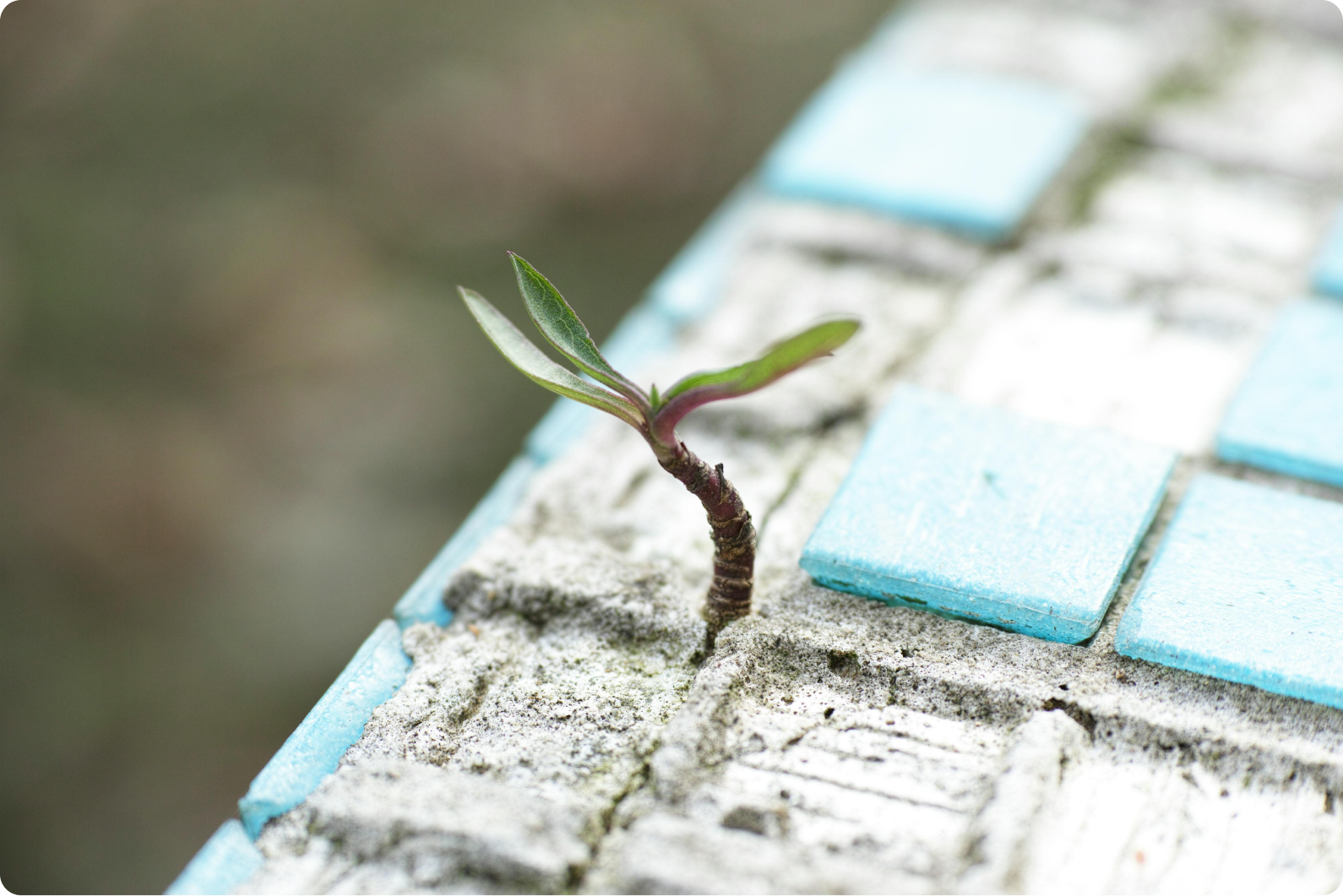
This guide explores what low-carbon concrete is, how it can be produced, and why decarbonizing concrete is crucial for reducing the embodied carbon footprint of the built environment.
Why low-carbon concrete is the road to net-zero
Concrete is the most consumed man-made material in the world with 14 billion m3 of concrete produced in 2020. Concrete is a critical construction material for buildings and infrastructure projects. Its popularity is due to its strength, durability, versatility, and in most cases relatively low cost.
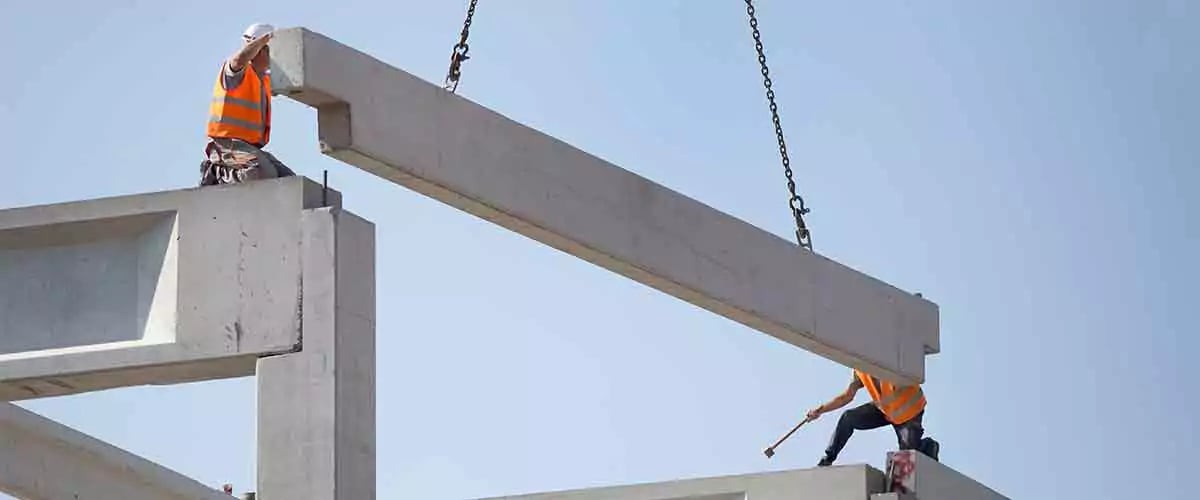
Problems with concrete
Concrete comes with a high embodied carbon impact, which is caused by the production of cement, one of its main constituents. This is due to cement’s energy-intensive production process and carbon emissions from the chemical calcination process. Another challenge when using concrete is reusability. Practically all concrete today is crushed into aggregates at the end of the building’s life. Due to the very high volume used, there’s no material that can replace the use of concrete. While alternative construction materials have a valuable role in the decarbonization of the built environment, their scalability is not sufficient to replace concrete. Other materials however help spur innovation in the concrete sector to improve its environmental performance.
A quick guide for design teams and contractors |
A checklist for reducing embodied carbon when building with concrete:
|
Why is concrete so carbon-intensive?
Concrete is made of cement, water, sand, aggregates, and, in some cases, chemical admixtures for increased workability. Most of the concrete’s carbon emissions come from cement. Cement, in turn, consists of clinker (the traditional Portland cement) and other materials. Clinker is made by heating crushed limestone, clay, and other materials in temperatures as high as 1400 degrees C. This temperature can only be achieved with high energy content fuels, so most of its production relies on fossil fuels. In these temperatures, limestone (CaCO3) is decomposed into lime (CaO) and CO2. The resulting process carbon dioxide is referred to as calcination carbon. The calcination process is necessary for the clinker to function as a binder, so avoiding it is impossible. Very often, the clinker is mixed with other materials to achieve the desired properties for the cement.
Concrete is often used in monolithic structures, or cast in place, which does not allow it to be easily reused after a building’s end of life. The most circular end-of-life scenario is for concrete to be crushed into aggregates. Meaning that new concrete is needed for future buildings with all the additional carbon emissions that come with it.
Figure 1: The typical composition of traditional concrete |
Figure 2: The cement manufacturing process: Cylinder lime-burning kiln |
What is low-carbon concrete?
What is broadly perceived as a low-carbon concrete in the construction industry, is a concrete mix that results in lower embodied carbon compared to an average concrete mix. However, there is currently no globally agreed definition for low-carbon concrete.
Challenges with low-carbon concrete
- There is no agreed benchmark in most regions and no agreed percentage reduction of embodied carbon in order for the concrete mix to be classified as low-carbon.
Local attempts include the Concrete Sustainability Council (Germany), Lavkarbonbetong standard (Norway), and the UK’s Low Carbon Concrete Routemap. This results in the term being used in any case where concrete has lower embodied carbon than a typical Portland cement mix, even if the reduction is minimal and at times even nominal. In most cases, the reduction of embodied carbon will be the result of cement substitution with more traditional alternative binders like Fly Ash, Ground Granulated Blast Furnace Slag (GGBS), calcined clays and in some limited cases natural pozzolans, as well as with innovative new solutions. This is not an exhaustive list, many other solutions exist and new ones are being developed.
- The problem with most of the low-carbon concrete made today is that clinker (the key ingredient of cement) is substituted with secondary materials from fossil fuel-based processes. While this is a useful transition mechanism, it cannot scale as we decarbonize power generation and other industries.
Benefits of reducing cement clinker
1. Reduced embodied carbon from concrete
Concrete is used in foundations, slabs, and the structural frame of most buildings across the world. With the majority of concrete’s embodied carbon coming from the production of cement the key to reducing concrete’s embodied carbon is to reduce the total amount of cement used. This can be done via:
- Avoid overdesign of structural elements: this will reduce the amount of concrete and cement being used.
- Rationalize the consideration of live loads during design: live loads are often overestimated, which can be avoided through detailed structural design without compromising the building's future adaptability.
- Design for material efficiency: optimize the span of the structural grid and incorporate more material efficient concrete elements like hollow core slabs, and composite decks.
- Avoid over-specifying concrete’s compressive strength: concrete mixes can be optimized for specific parts of the building; there’s no need to use standard strength throughout.
- Avoid over-specifying concrete’s compressive strength within the first 28 days after pouring: this allows the use of concrete with alternative binders that in most cases have longer curing times.
- Use alternative binders: try using Fly-Ash, GGBS, calcined clays, etc.
- Use other innovative concrete solutions: when available, try using solutions that are not necessarily related to cement reduction, such as high-performance concrete (HPC), fiber-reinforced concrete, or pervious concrete.
2. Cutting costs for concrete producers
A key benefit of optimizing concrete and cement clinker quantity is that it saves capital costs. By reducing the amount of clinker used in concrete production, manufacturers can decrease their reliance on expensive raw materials and energy-intensive processes. This not only lowers production costs but also enhances the overall efficiency of the manufacturing process. Additionally, using alternative binders and optimizing the concrete mix design can lead to reduced waste and improved resource utilization. This cost-saving potential is especially significant for large-scale construction projects, where even small reductions in material usage can translate into substantial financial savings. Moreover, adopting low-carbon concrete practices can improve a company's market competitiveness and align with increasing regulatory and societal pressures for more sustainable construction methods.
Alternative binders to cement
The most commonly used alternative binders to cement are:
- Fly Ash / Pulverized Fuel Ash
- Ground Granulated Blast Furnace Slag
- Calcined Clays
Although some are widely used by the construction industry, their availability depends on fossil fuel-related processes and is expected to be limited in the future when it is hoped that fossil fuel use will decrease. As a result, the use of such binders is important today but cannot be a significant part of concrete’s zero-carbon future.
Challenges from alternative types of cement binders
Although the binders listed above will reduce concrete’s embodied carbon, they are often viewed with skepticism by contractors since they affect the curing rate of concrete. This means that concrete mixes with such binders will need more time to reach a set level of strength compared to traditional Portland cement mixes. A concrete mix with 50% GGBS for example will have half the strength of a Portland cement mix in the 7 days after pouring but should reach the same strength within 28 days. The slower curing time can hold construction back by a few days for each building floor added which can result in bigger delays and cost implications in taller building constructions. Contractors will have to plan for the longer curing time of low-carbon concrete where possible to mitigate the delays and associated cost implications.
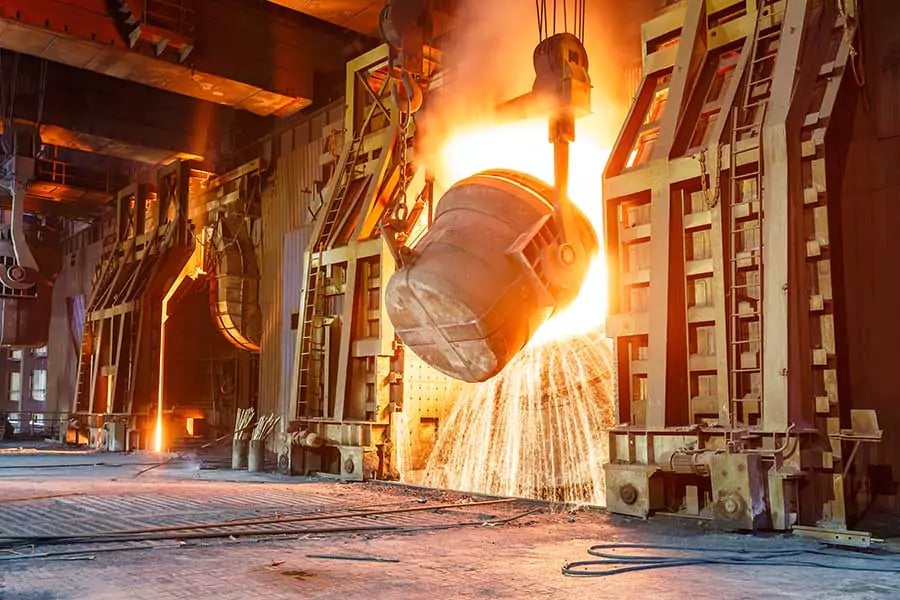
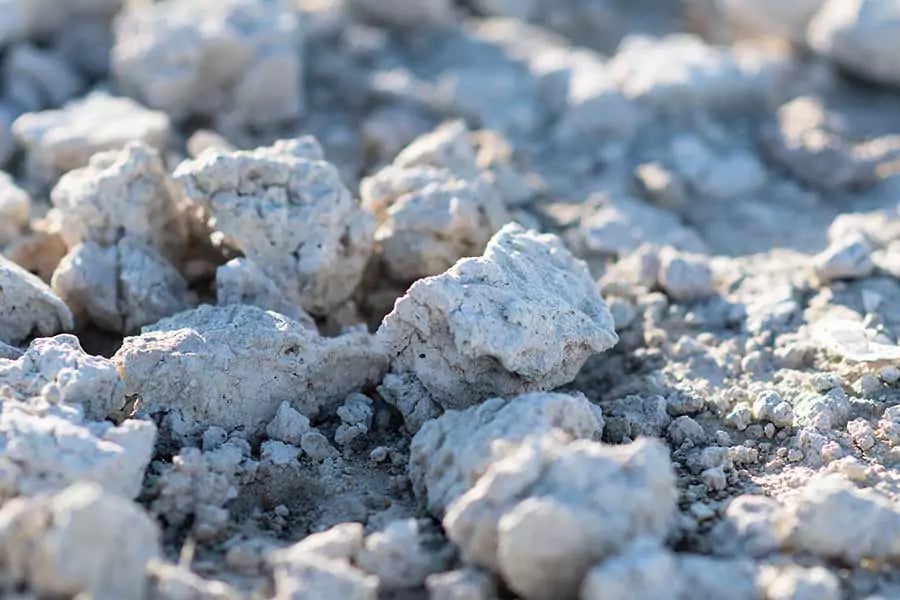
Other binders to cement
Other alternative binders, albeit not used as extensively, include:
- rice husk ash
- gypsum
Rice husk ash is a by-product of rice production. When rice husks are removed from rice, they are burned. The ash resulting from this process is known as rice husk ash and has a high content of silica which allows it to produce additional cementitious compounds when reacting with carbon hydroxide which is a product of cement hydration. A key benefit of rice husk ash is that it is a renewable resource.
Alternative low-carbon concrete solutions
So, is low-carbon concrete all about alternative binders to cement? No, other low-carbon concrete technologies are now emerging with some already commercially available and scaling up.
-
Carbon capture technologies
A significant cause of the carbon emissions of concrete is the calcination process during the production of cement. Even if the main energy being used in the production and transportation of concrete were zero carbon, the calcination-related emissions would remain the same. To address this, Heidelberg Materials is currently building the world’s first carbon capture and storage facility in a cement production plant in Brevik, Norway, and is planning to do the same in the Slite plant in Sweden.
-
Carbon Dioxide injection
Such technologies aim to use captured CO2 by injecting it into concrete while still in liquid form. CO2 then reacts with cement and water to produce more cementitious compounds. Such technologies already exist commercially and can be applied to existing concrete plants. CarbonCure, a Canadian company, and Carbonaide, a Finnish company, are developing technologies that can be used in existing ready-mix concrete and precast concrete respectively. CarbonBuilt has developed a similar technology for the production of ultra low-carbon concrete blocks. The technology can be integrated into any existing concrete block plant and reduces the embodied carbon of the blocks by replacing the cement with another alternative material that reacts with injected CO2 during the curing process to form CaCO3 (limestone). The first commercial production of concrete blocks using this technology was started by Blair Block in Alabama, USA, in 2022.
-
Biotechnology
Research is also being undertaken on how low-carbon concrete can be achieved with the use of biotechnology. Prometheus Materials in the US has developed a concrete mix that uses algae to replace Portland cement. Their solution has been used in pilot projects and efforts are being put towards the full commercialisation of the product. Biozeroc, a startup in the UK is a biomaterials company that produces low-carbon concrete using bacteria. Their process uses bacteria to bind aggregates and sand together into a material that performs as well as conventional concrete. This BioConcrete production process emits at least 85% less carbon and has the potential to be carbon-negative once waste materials are incorporated into the bacterial feedstocks.
-
Plant-based solutions
Plant-based solutions include materials that in many cases are a mix of lime, sand, clay, and plant fibers. One such material is hempcrete which is made of lime, sand, and hemp fibers. Such materials can be used for cast-in-place elements, shotcrete, and masonry units. Although these materials are bio-based and come with ultra-low embodied carbon, they cannot substitute traditional concrete other than for small buildings and non-load-bearing applications.
How to make concrete more circular
The role of specifiers and contractors
To reduce concrete’s embodied carbon, which is primarily released during the production stage (A1-A3), focus on minimizing the need for new concrete production. This can be achieved by using reusable precast elements with reversible connections. Specifiers should adopt performance-based requirements, including durability, shrinkage, compressive strength, and embodied carbon limits. Contractors should procure low-carbon concrete using environmental product declarations (EPDs) where available, prioritize alternative binders, and reduce onsite wastage. Although alternative binders lessen the impact, significant A1-A3 emissions remain. Therefore, reusable concrete elements and minimizing new production are crucial for sustainable construction, aligning with low-carbon goals.
Who can supply low-carbon concrete solutions?
Many manufacturers are already producing low-carbon ready-mix and precast concrete or cement. All manufacturers that have created EPDs for their concrete mixes or cement products can be found and compared in the One Click LCA database.
Below is a list of low-carbon concrete manufacturers for reference:
Europe |
North America |
Middle East and North Africa |
|
|
|
Leveraging One Click LCA’s EPD Generator to create EPDs for concrete
With One Click LCA’s Concrete EPD Generator, concrete and cement manufacturers can create environmental product declarations (EPDs) for their mixes at a fraction of the effort and cost compared to traditional EPD solutions. The tool generates EPDs that are third-party verified and comply with ISO 14025, EN 15804+A2, and associated standards, and it unlocks the market for more products to demonstrate their sustainability credentials.
Concrete EPD generator tool
Create & publish EPDs for your ready-mix concrete
For concrete manufacturers to create and publish reliable, third-party verified concrete EPDs — fully compliant with global EPD standards.
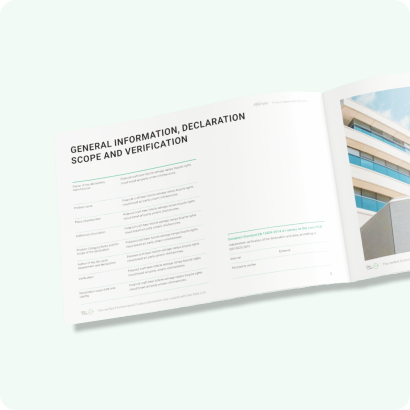